6G, the sixth generation of mobile networks, promises to transform wireless connectivity for operators, consumers and businesses. Now that the initial framework and goals of 6G have been defined, it is now entering into its pre-standardization phase, with labs and researchers evaluating and testing technical capabilities that aim to deliver on its objectives. Given that the target for commercialization is from 2030, the window for evaluating and creating capabilities is relatively short. This blog therefore explains more about 6G and its benefits and provides a deep dive into specific technological pillars and how they can be effectively tested and developed in the lab.
Now that 5G is increasingly prevalent in mobile networks across the globe, the technology and development focus is shifting to 6G. This looks to build on the capabilities of 5G to integrate emerging technologies that improve performance while minimizing the environmental impact of networks.
In terms of timescales, the International Telecommunication Union (ITU) defined the initial framework and goals for the development of 6G under the ITU-R M.2160 recommendations in 2024, and the objective is to deploy first applications and commercial networks from 2030.
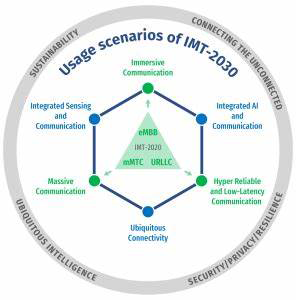
Figure 1: 6G scenarios and capabilities (source ITU)
As can be seen in figure 1 above, 6G extends 5G (the central triangle in the graphic) through six usage scenarios:
- Immersive communication to deliver augmented throughput
- Massive communication to handle a larger number of connectable and connected objects
- Hyper reliable and low-latency communication to ensure greater reliability and reduced latency
- Integrated sensing and communication to enable new use cases based on environmental detection capabilities
- Artificial Intelligence and communication capabilities that add native artificial intelligence to the network
- Ubiquitous connectivity that offers constant, always-on communication
Four prime characteristics are used as key design principles for all usage scenarios. These are shown as the outer circle of the figure 1 graphic.
- Sustainability to guarantee optimal use of energy and natural resources, such as via energy-aware network design, dynamic power management, and green infrastructure
- Security and Resilience to deal with confidentiality and data integrity for users, along with the ability to provide quick connectivity service recovery from any disruptive events
- Connecting the unconnected to reduce the digital divide through affordable network deployment and connectivity services
- Ubiquitous intelligence to integrate intelligence as well as interoperability into every aspect of communication networks
Delivering these usage scenarios requires new capabilities (figure 2), whose values and targets will be defined in the pre-standardization phase running up to 2027. These include wider coverage, more precise location positioning, and AI, sustainability, and interoperability capabilities.
Capabilities of IMT-2030
Note: The range of values given for capabilities are estimated targets for research and investigation of IMT-2030.
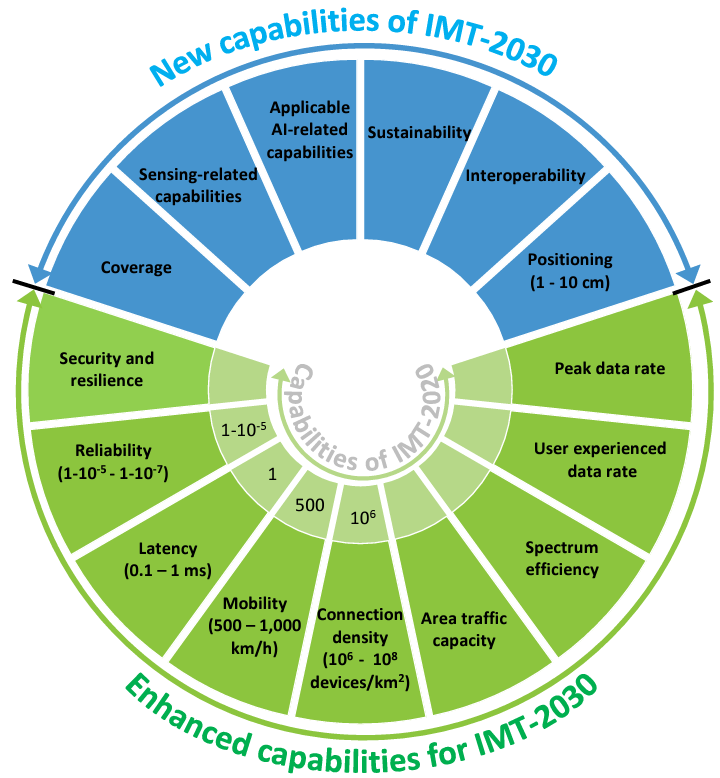
Figure 2: ITU-2030 capabilities (Source ITU)
The 6G standard will rely on key technologies to deliver its capabilities and performance. Equipment providers’ and operators’ innovation and strategy teams, as well as research and technology organizations (RTOs), are currently researching and testing these technological pillars through virtual lab testing ahead of commercialization, a process that is vital to proving and patenting key innovations.
Successful testing requires high-fidelity prediction models, accurate channel simulation and the ability to undertake complex environmental modeling (both indoor and outdoor) in a virtual lab, such as a telecom digital twin. This enables organizations to assess performance in future scenarios, while balancing key factors such as capacity, complexity, cost, and sustainability.
In this section of the blog, we’ll deep-dive into some of the most innovative areas within the 6G RAN (Radio Access Network), and how they can be developed and tested.
6G technology is expected to integrate AI into every facet of how 6G networks operate, such as optimizing the utilization of network resources, improving link performance, and automate infrastructure reconfiguration.
In the current phase of 6G development, organizations need to focus on designing machine learning models and AI algorithms to deliver optimal network resource management, improved security, reliability and latency and then create a framework for training, learning and testing these new solutions in the lab across a wide variety of scenarios.
Effective testing requires the generation of synthetic channel data to define networks, create effective digital twins and train AI models. In this use case, the telecom RF digital twin is a virtual replica of a 6G network, used to test solutions in the lab by simulating realistic 6G networks. It is therefore vital that synthetic data used is accurate, realistic, and usable to guarantee accurate results that will then correctly predict and train models and algorithms.
A key step forward for 6G is the ability to seamlessly integrate (traditional) terrestrial networks with equivalent NTNs (such as those that use satellites, High-Altitude Platforms, and Unmanned Aerial Vehicles).
All of these networks should be accessible through the same device, without requiring additional hardware or device reconfiguring to provide “anything, anytime, anywhere” connectivity. This will open up new opportunities and use cases, such as in remote areas or in disaster recovery scenarios when terrestrial networks are out of action.
As well as supporting the “connecting the unconnected” characteristic of 6G, integrating NTNs can also increase sustainability, as network equipment is often solar powered, although the process of initially launching satellites is energy-intensive.
At a technical level, the key issue that needs to be tested and perfected is around handover between terrestrial and NTNs, which is difficult given that NTNs are deployed through moving platforms such as satellites, planes, rather than being static. Additionally, equipment has to minimize interference when operating in the same or adjacent frequency band to optimize quality.
While still being researched, metamaterials can be used to accurately and dynamically reflect electromagnetic waves in any desired direction.
The use of Reconfigurable Intelligent Surfaces (RIS) is one promising application of metamaterials. RIS are composed of passive metamaterials and can be deployed externally on building façades or on walls indoors to reflect electromagnetic waves towards the user equipment direction, enhancing signal strength and quality. The aim is to improve signal reception in spaces where it has traditionally been poor such as deep indoors, in highly cluttered areas or when operating at bands within Frequency Range 2 (24.25 GHz to 71.0 GHz.)
As the name suggests, RIS can be controlled and dynamically reconfigured to change propagation conditions by reflecting or refracting signals, while using little or no additional power. They can therefore be efficiently combined with beamforming at the base-station or to enhance the performance of multiple-input multiple-output (MIMO) systems by creating favorable propagation conditions. This leads to higher data rates, increased quality and improved spectral efficiency, all while lowering overall power consumption and boosting sustainability compared to alternative deployments.
To move forward with RIS, operator labs to be able to create a model to simulate changing propagation conditions in real-world environments, moving it from theory to practice. They must be able to undertake precise and reliable performance evaluations, optimize signal propagation and reduce interference to successfully test the technology.
Metamaterials can be also employed to create a transmit array at the base-station that forms multiple beams in a more efficient way than conventional antennas, together with lower energy consumption.
Alongside metamaterials, new generations of MIMO systems are being investigated such as extremely large antenna arrays or distributed antenna systems. The first approach will offer increased capacity and might benefit from the interesting properties of the near-field communication range, while the second approach is thought to provide more uniform high-data rate coverage.
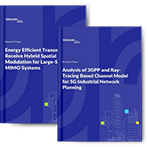
Driven by a passion for research and development, Siradel actively contributes to pioneering projects that advance the telecom industry, highlighting our role in shaping the future of connectivity.
ISAC – or JCAS (Joint Communication and Sensing) – is a pivotal technology for 6G networks, supporting the objective of merging communication and sensing functionalities into a unified system, removing the need for a separate sensing network.
ISAC should therefore enable 6G networks to “sense” the physical world, providing advanced, radar-like location-aware services and environmental monitoring capabilities. This could be used by drones in dense urban areas or robots/automated guided vehicles in factories. These objects need to be able to sense their position and reconstruct their environment (such as walls) to enable navigation. Additionally, ISAC has to overcome challenges around protecting the privacy/confidentiality of sensed data and offer the ability to combine data from multiple sensors and cameras. The algorithms to implement ISAC have to be tuned through testing in order to successfully deliver these capabilities.
6G frequency bands have not yet been standardized, with ongoing research happening in areas such as subTHz, FR3 band (7-20 GHz), and reusing old bands (such as 2G). The aim is to make more efficient use of available radio spectrum.
However, the current lack of standardized frequency bands poses a major challenge for equipment manufacturers and operators. They need to be able to ensure that their planned equipment operates effectively whatever bands are chosen – for example, high frequencies require larger antenna arrays.
Additionally, research labs need to be able to simulate MIMO performance in new frequency bands, with equipment that could be based on new MIMO architectures, new deployment strategies or signal processing schemes and then evidence the results for standardization purposes.
6G aims to incorporate moving objects into the network, whether terrestrial users, such as cars (Vehicle-to-everything/V2X) or trains, or moving cells via NTNs, with antenna on satellites or drones acting as receivers/transmitters. These applications require ultra fast data transfer, low latency, and high reliability – capabilities that are complex to achieve due to range of networks and their motion, requiring dynamic connectivity.
Labs need to be able to model this complex environment to understand and test dynamic scenarios, especially in use cases
We are currently at a key stage in the 6G journey with research ongoing into its technological pillars before performance metrics are set around key capabilities. This makes it crucial for research labs to be able to effectively and accurately test and realistically simulate multiple scenarios with high precision, in order to cover all possibilities and provide evidence ahead of standardization processes. Organizations therefore need to harness effective testing and simulation technologies, backed by experience and expertise from partners who understand the space and can help them achieve their goals. At Siradel we’re trusted by leading equipment makers and operators, as well as being part of multiple research projects. Contact us to discuss how we can help you deliver on your 6G testing needs.